ADVANCEMENTS IN MICROFLUIDICS FOR HEALTHCARE
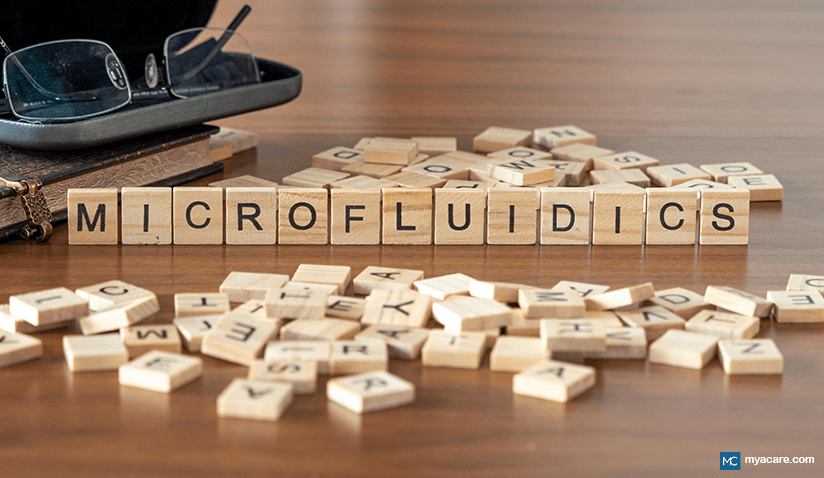
Medically Reviewed by Dr. Rae Osborn, Ph.D. - September 11, 2024
Advantages of Microfluidics in Healthcare
Different Types of Microfluidic Devices
Microfluidics is a rapidly growing field that has revolutionized how we approach healthcare. It involves the manipulation of small amounts of fluids, typically on the scale of microliters or nanoliters, in miniscule channels or chambers built into a microchip. This technology has enabled the development of miniaturized devices that can perform complex tasks, making them valuable tools in various healthcare applications.
This article explores the advancements in microfluidics and their impact on personalized medicine, diagnostics, drug discovery, and other healthcare applications.
How Does Microfluidics Work?
Microfluidic devices are small inventions that control the flow of fluids through minute channels and chambers on a microchip. A computer interface records and analyzes the fluid's response, revealing a lot about the material's nature. This setup allows researchers and scientists to quickly run tests and experiments to identify various biological fluids and substrates and understand their unique properties.
Microfluidics can help to diagnose disease, infection, and contaminants at the cellular (microscopic and nanoscopic) level. It is currently revolutionizing the design of drug delivery systems and can emulate microscopic tissue environments for advanced lab testing.
Materials and Manufacture
A broad spectrum of microfluidic devices is available, and a lot are under development. These devices use various materials, including glass, silicon, polymers, hydrogels, ceramics, and microelectronic components[1]. Paper is also a potential substrate for microfluidic devices, which may rapidly reduce production costs.
The channels and chambers are etched onto the chip using photolithography, soft lithography, or laser ablation techniques. These manufacturing methods allow for the invention of very sophisticated channel patterns or perforations for fluids to run or drop through. Electrical components integrate into microfluidic devices that can sense the movement of the fluids, measure the size of droplets, and record various other properties, such as surface tension and how particles in a fluid respond to an electric current.
The design of the channels and chambers varies according to the device’s application. They all typically have dimensions in the range of micrometers and nanometers.
Photolithography is a cornerstone technique for getting microfluidic devices sized to this range. Photolithography is a specific manufacturing technique that molds polymer sheets into a desired pattern using UV light and a stencil called a mask to guide the process[2].
Microfluidic Techniques
Microfluidics can be divided into three categories: continuous-flow microfluidics, droplet-based and digital microfluidics[3].
- Continuous flow microfluidics utilizes a constant and regular liquid flow through microchannels. Devices like external pressure pumps or integrated mechanical micropumps manipulate the flow. This flow type has many applications, including bioanalytical, chemical, energy, and environmental fields.
- Droplet-based microfluidics, is the movement of droplets in channels using immiscible fluids.
- Digital microfluidics, also known as emulsion science, manipulates autonomous droplets on a substrate using electro-wetting. This technique allows for generating and controlling uniform, reproducible droplets throughout various experiments. Digital microfluidics' applications include nanoparticle synthesis, single-cell analysis, and encapsulation of biological entities.
Acoustofluidics, optofluidics, and electrophoresis are subcategories of microfluidics that utilize specific phenomena to manipulate fluids at the microscale. They can integrate with continuous flow and digital microfluidics to achieve specific functionalities.
- Acoustofluidics leverages sound waves to manipulate fluids. This approach has various applications, including particle manipulation, cell sorting, and mixing in continuous and digital microfluidic devices.
- Optofluidics employs light to control fluids. It finds applications in particle trapping, biosensing, and manipulating droplets in continuous and digital microfluidic systems.
- Electrophoresis, or electrokinetics, utilizes an electric field to separate or move charged molecules or particles. It commonly integrates with continuous-flow microfluidics for applications like DNA and protein separation, among other specific purposes.
Other techniques can combine with microfluidic devices to emulate sophisticated laboratory equipment. These include:
- Fluorescence Spectroscopy and Micro-Scintigraphy: Microfluidic channels can accurately control the flow of fluids containing fluorescently labeled molecules[4]. Researchers can gain information about their concentration, binding interactions, and other properties by analyzing the emitted light from these molecules.
- Surface Plasmon Resonance (SPR): Microfluidic chips can merge with SPR sensors for precision molecule detection. These sensors use light to glimpse differences in the refractive index of the fluid’s surface caused by biomolecule interactions. This sensitivity allows for label-free detection and characterization of biomolecules in the fluid.[5]
- Microscopy: Light microscopy can help image and analyze cells, particles, or other suspended elements within the microfluidic channels. The precise control over flow offered by microfluidics allows for high-resolution and dynamic microscopic analysis.[6]
Advantages of Microfluidics in Healthcare
Microfluidic devices have several advantages over traditional methods in healthcare.
The devices' small size allows for high throughput and single-cell analysis, making it ideal for personalized medicine. The devices are also highly sensitive, allowing for the higher-resolution detection of single molecules or cells.[7]
Additionally, microfluidic devices are cost-effective, require small sample volumes, provide greater control over samples, and can easily merge with other technologies, such as biosensors and imaging systems.
Different Types of Microfluidic Devices
Various types of microfluidic devices are used in healthcare, each with its unique design and function. Some of the most common types include:
Lab-on-a-chip
Lab-on-a-chip (LOC) devices integrate multiple laboratory functions onto a single chip, often just millimeters or centimeters in size. These devices can perform sample preparation, mixing, separation, and detection, offering advantages in portability and rapid analysis. LOCs find widespread applications in point-of-care diagnostics, drug discovery, environmental monitoring, and even biochemical analysis in space missions due to their minimal resource requirements.[8]
Microfluidic Organ-on-a-Chip and Bioreactors
Organs-on-a-chip technology offers a powerful way to model human organs in a miniature, controlled environment. These devices use microfluidic channels to replicate physiological conditions, house living cells, and mimic the flow of fluids within a target organ. Bioreactors provide a similar but larger-scale environment for growing cells in a controlled setting.[9]
They present advantages over traditional cell culture and animal models by providing a more realistic and human-relevant platform for studying diseases, testing drugs, and potentially creating personalized medicine solutions.
Microfluidics in Diagnostics
Microfluidics has had a significant impact on diagnostics. They allow for rapid diagnosis and early identification of diseases, improving patient results and reducing healthcare costs.
Microfluidic devices have enabled the development of point-of-care tests for diverse diseases, such as infectious illnesses, cancer, and genetic disorders.
These devices can achieve this through micro purification of DNA from cells and integrating with technologies such as PCR (polymerase chain reaction testing) or spectrophotometry. They also have great potential for micro-imaging, enabling scientists to watch the movement of radioactive tracer molecules through cells or biological fluids, when combined with radioluminescence microscopy.
More frequently seen applications of microfluidic point-of-care devices include blood glucose testing and pregnancy kits.
Some pivotal applications of microfluidics in diagnostics include:
- Point-of-Care Diagnostics: Point-of-care diagnostics refers to tests that can be performed at the patient's bedside, doctor's office, or even at home. These tests are typically rapid and require minimal sample preparation, making them ideal for resource-limited settings.
- Rapid Diagnosis: Microfluidic devices can perform tests in minutes, making them useful for rapid diagnosis. Speed is particularly critical in emergencies where quick and accurate diagnosis is crucial for patient care.
- Early Disease Detection: Swift diagnosis is essential for successful treatment and improved patient outcomes. Microfluidic devices can detect biomarkers, such as proteins or nucleic acids, at very low concentrations, allowing for the early detection of diseases such as cancer and infectious diseases.
- Onsite Genetic Testing: Microfluidic devices play a role in the development of onsite genetic testing, allowing for real-time detection of genetic mutations and diseases. In the future, this can revolutionize genetic counseling and prenatal testing.
Microfluidics in Drug Discovery
Microfluidics has transformed the drug discovery process, making it faster, more efficient, and cost-effective.
High-throughput screening refers to batch testing large numbers of compounds for their potential as drugs. Microfluidic devices can perform high-throughput screening at a fraction of traditional methods' time, space requirements, and cost. With this technology, researchers can simultaneously screen for many more drugs in a higher degree of substrates. The devices can be automated for even faster results.
This rapid screening is not limited to testing novel active ingredients in fluids. Organ-on-a-chip devices that mimic the structure and function of human organs can do away with the need for profuse animal testing, complicated lab environments that aim to keep organs alive in jars, or computer simulations prone to inaccuracy.
The refinements these devices impart to drug delivery have also revolutionized drug discovery by changing the properties and efficacy of older agents.
Microfluidics endow drug delivery systems with precise micro-nano fluid particle mechanics. These devices can deliver drugs to specific locations in the body with a much higher accuracy than previous methods. They can also control the release of drugs, making them useful for targeted drug delivery and reducing side effects.
Lastly, microfluidic devices can produce nanoparticles enhancing medications and delivery systems. Examples include liposomes and lipid nanoparticles, nanoparticles made of polymers, metals, and minerals, quantum dots, and hybrid nanoparticles.[10]
Other Applications of Microfluidics in Healthcare
Microfluidics has an expansive range of medical applications beyond diagnostics and drug discovery.
Some other areas where microfluidics is impacting include:
Tissue Engineering: Microfluidic devices excel in tissue engineering by offering precise control over the cellular microenvironment. They regulate the flow of nutrients, oxygen, and signaling molecules, promoting the development of complex, functional bio-synthetic tissues. Microfluidics also allows for the creation of organoids – miniature versions of organs, useful for disease modeling and drug testing.
Regenerative Medicine: Regenerative medicine leverages cells, tissues, or organs to repair or replace damaged tissues. Microfluidic devices offer significant benefits in this field:
- Cell Culture and Expansion: Microfluidic platforms provide tailored environments for culturing and expanding stem cells or other therapeutic cell types.
- Cell Delivery and Encapsulation: Microfluidics can help create biocompatible scaffolds or microcapsules for controlled delivery and protection of regenerative cells to the target site.[11]
- Drug and Therapy Screening: Microfluidic "organs-on-a-chip" are useful for investigating the efficacy and safety of regenerative therapies, including stem cell treatments, growth factors, and precisely targeted nanoformulated drugs.[12]
- Immunoisolation: Recent developments suggest microfluidics could enable immune-isolating capsules for transplanted cells, potentially reducing rejection risks.[13]
Telemedicine: Telemedicine is the use of technology to dispense remote healthcare services. Microfluidic devices are revolutionizing telemedicine by enabling remote sample collection, monitoring, and analysis.
For instance, patients could use disposable microfluidic chips to self-monitor blood glucose levels, essential for diabetes management. These chips can wirelessly transmit results to healthcare providers, facilitating remote insulin dosage adjustments. This integration of microfluidics in telemedicine offers convenience and improved health monitoring for patients with chronic conditions.[14]
Latest Advancements and Future of Microfluidics in Healthcare
Microfluidics is a rapidly evolving field, and several recent advancements have further improved its capabilities.
Integrating microfluidics with other technologies, such as biosensors, imaging systems, and AI, has paved the way for next-generation diagnostics. AI can analyze large amounts of data generated by microfluidic devices, providing insights that can improve patient care and drug discovery.
These devices can provide real-time, accurate, and personalized diagnostics, improving patient outcomes and reducing healthcare costs. In the future, microfluidic devices can allow for personalized health advice based on the patient's current biomarkers, taken at home or the doctor's office.
Two of the most cutting-edge applications include:
- Microfluidic Biosensors: Microfluidic biosensors combine the sensitivity of microfluidics with the specificity of biosensors, allowing for the detection of specific biomarkers or cells in a sample. This technology has the potential to revolutionize wearable devices. Wearable sensors, already used for continuous glucose monitoring and physiological measurements, will eventually integrate microfluidics. This modification will allow smartwatches and other devices to read and analyze biological samples and data efficiently, providing information to guide personal or clinical decisions.
- Implantable Nanofluidic Devices: Incorporating nanotechnology into microfluidic devices allows for the manipulation of particles and cells at the nanoscale. This collaboration has enabled the development of implantable microfluidic devices for improved drug delivery and biosensing. One study shows how a nanofluidic implantable device can deliver CD40 antibodies to mice with pancreatic cancer, lowering tumor volume and cutting the therapy dose down by four times.[15]
- Self-Powered Devices: Microfluidic devices could potentially harvest energy by converting the movement of ions (charged particles) in a flowing liquid into electricity. This might involve using microchannels to create specific flow patterns or incorporating materials that interact with the ionic current to generate a voltage. In the future, these devices will power themselves based on these aspects of fluid dynamics.[16]
Challenges Faced in Implementing Microfluidics in Healthcare
While microfluidics can transform healthcare, some challenges still need to be addressed for its widespread adoption. Some prime challenges include:
- Cost: The fabrication of microfluidic devices can be expensive, hindering adoption by smaller companies and research labs.
- Standardization: Lack of standardization in design and fabrication creates challenges regarding the interpretation of results across different studies involving microfluidic devices.
- Integration with Existing Technologies: Integrating microfluidic devices with technologies like biosensors and imaging systems can be complex and demands specialized expertise.
Conclusion
Microfluidics has significantly impacted healthcare, and its potential for further advancements is immense. From personalized medicine to drug discovery, microfluidics revolutionizes the global approach to healthcare. With continued research and development, microfluidics has the potential to drastically enhance patient care, reduce healthcare costs, and pave the way for precision medicine.
To search for the best doctors and healthcare providers worldwide, please use the Mya Care search engine.
Sources:
- [1] https://www.ncbi.nlm.nih.gov/pmc/articles/PMC7921936/
- [2] https://www.researchgate.net/figure/Photolithography-to-fabricate-microfluidic-devices-A-A-photoresist-is-spin-coated-on_fig3_258010193
- [3] https://www.researchgate.net/figure/The-comparison-between-the-three-types-of-microfluidics_tbl2_285546332
- [4] https://www.horiba.com/int/scientific/technologies/fluorescence-spectroscopy/what-is-fluorescence-spectroscopy/
- [5] https://www.ncbi.nlm.nih.gov/pmc/articles/PMC5017341/
- [6] https://www.ncbi.nlm.nih.gov/pmc/articles/PMC8877635/
- [7] https://www.news-medical.net/life-sciences/What-is-Microfluidics.aspx
- [8] https://www.frontiersin.org/articles/10.3389/frlct.2022.979398/full
- [9] https://www.mdpi.com/1422-0067/24/4/3232
- [10] https://www.mdpi.com/2673-8023/3/4/58
- [11] https://www.ncbi.nlm.nih.gov/pmc/articles/PMC8773546/
- [12] https://www.ncbi.nlm.nih.gov/pmc/articles/PMC7975637/
- [13] https://news.mit.edu/2024/scientists-develop-low-cost-device-safer-cell-therapy-0207
- [14] https://ieeexplore.ieee.org/abstract/document/6705436
- [15] https://www.news-medical.net/news/20230413/Using-an-implantable-nanofluidic-device-to-tame-pancreatic-cancer.aspx
- [16] https://www.researchgate.net/publication/336486207_Ion_Transport_in_Nanofluidic_Devices_for_Energy_Harvesting