RECENT DEVELOPMENTS IN X-RAY IMAGING: ADVANCED COMPUTATION TO PORTABLE DEVICES
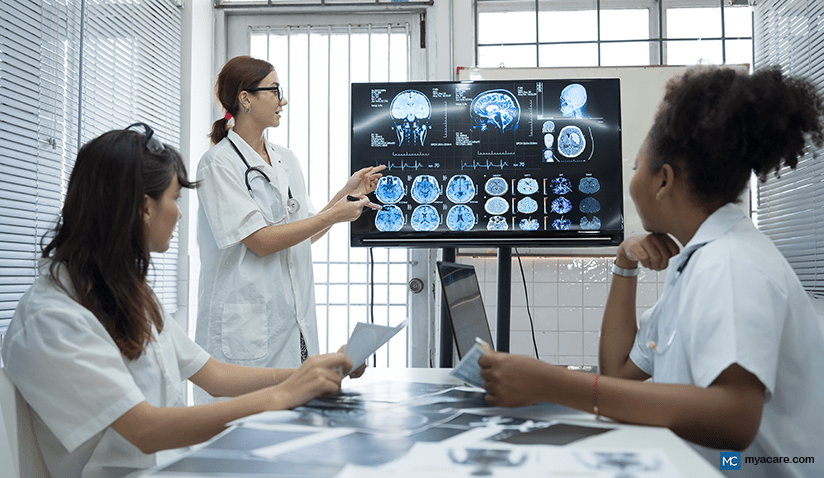
Medically Reviewed and Updated by Dr. Sony Sherpa (MBBS) - October 04, 2024
Since its discovery, X-ray imaging has rapidly changed the face of diagnostics and treatment over the last century for a wide variety of health conditions. With recent advances, the ability to non-invasively see through objects in high-definition detail is making science fiction an imminent reality.
The below article explains how X-ray imaging works and covers the latest advancements in X-ray imaging technology.
What Are X-Rays?
Similar to light waves, X-rays are a form of electromagnetic radiation. Like light, they carry photons. However, X-ray photons hold more energy than ordinary photons. When X-rays bombard matter, including body tissues, some of the electrons in the bombarded atoms are temporarily knocked out of their orbit by X-ray photons. This displacement can be captured on camera in the brief moment it occurs, allowing the X-ray photons to illuminate the structures it bombards.
Hard body tissues such as bone show up lighter on X-rays due to having larger atoms capable of absorbing a higher amount of X-ray photons upon bombardment. Soft tissues have smaller atoms, showing up as black or grey on X-ray scans due to less photon absorption. Contrast medium injections into soft tissue can enhance its ability to absorb X-ray radiation and show up better on X-ray photographs.[1]
The History of X-Rays and X-Ray Imaging
X-rays were discovered by accident in 1895, thanks to the experiments of Wilhelm Roentgen, a German physicist. This happened shortly after the invention of the first cathode tubes, known as Crookes tubes.[2]
Basic Mechanism of Cathode Tubes. Crookes tubes were foundational to all the X-ray technology we use today. They comprise two ends of a high-voltage (up to 100 kV) battery connected to a partially de-pressurized gas chamber. Electrons are generated and accelerated from the battery due to the high current. These collide with the gas inside the chamber to produce beams of light. Magnets can be used to change the direction of the light inside the chamber.
The Discovery of the X-ray. When experimenting with these early cathode tubes, Wilhelm noticed that objects placed in the path of the weak electron beam lit up in a similar way to the gas particles in the chamber. He eventually placed his hand in the way of the beam and saw his bones reflected on the black backdrop. Expansions on his experiments revealed that X-ray photons behave differently compared to ordinary photons as they are able to pass through objects, which was later explained to be the result of their increased energy potential and excitation.[3] Since their widespread adoption following this discovery in 1900[4], X-rays and X-ray imaging changed the face of science, physics, and medicine, forming the basis for many diagnostic and treatment tools today.
How Radiography Was Invented. Other than X-rays themselves, imaging is the other half of the equation with respect to X-ray-based devices. A few months after X-rays were discovered, it was realized that capturing radiographs worked in a similar fashion to photography. A film developed specially for capturing X-ray images placed behind the exposed tissue would capture the radiograph through particle displacement on the film’s surface. These days, film-screen radiography has been replaced by digital X-ray detectors in the majority of X-ray imaging applications.
Modern Developments in X-Ray Imaging Technology
X-ray imaging technology has become a lot more powerful, selective, and computerized.
X-ray imaging can be divided into two parts: the generation of the X-rays themselves and the technique involved in capturing and producing an X-ray image. Modern developments in these aspects are briefly explained below.
X-Ray Beam Generation and Focus
In order to generate an X-ray image, X-rays need to be generated and focused on the area of interest. As explained above, X-rays are generated through accelerating electrons, which displace other electrons in order to generate X-ray photons.
Modern techniques have substantially improved X-ray beam generation in the following ways:
- High-Voltage Alternating Currents and Electron Acceleration. Electrons are usually generated from a heat source and propelled using high-voltage electronic devices that alternate the current. The alternating current promotes electron acceleration, which increases their energy and the resultant energy of the X-rays produced by the device.
- Vacuum Guided Beam Enhancement. The electrons pass through a vacuum chamber, the likes of which are vastly superior to the original equipment used to create X-rays. They do not hit any gas on their way, allowing for an extremely concentrated beam of energy.
- Metal Use in X-ray Generation. Before exiting the acceleration track, the electrons are made to hit one or more sheets of metal. The electrons in the metal become displaced, generating X-ray photons that leave the device and pass through the body tissue or object of interest to be X-ray imaged. Only 1% of the electrons that hit the metal plate are able to generate X-rays.[5] The speed of the electron beam increases the number of X-rays produced, as well as their energy intensity, while the number and thickness of metal sheeting used decreases X-ray intensity.
- X-ray Diffusion Techniques. Like light, the resultant X-ray photons can be focused toward a single point (like a laser), spread out like a fan, or projected like a cone of light. These configurations describe the differences between distinct X-ray applications, with an X-ray laser often being used for surgery and radiation therapy. Cone beam and fan beam X-rays are used for precise modern imaging, often used in computerized tomography or CT scanning.
- Magnetic Beam Shaping. Magnets are often incorporated into modern X-ray machines to shape the beam, allowing the X-ray to reach a target more precisely and to be diffused accordingly.
Radiologists, dosimetrists, and medical physicists who operate X-ray devices take advantage of the radiological aspects mentioned above in order to maximize X-ray imaging. The beam intensity can be controlled on a digital interface that allows for optimal control and precision with respect to tissue depth and image resolution.
X-Ray Capture and Imaging Plates
Radiography refers to the capture of X-rays that have passed through a medium in order to generate an image. Imaging techniques have vastly improved due to the use of electronic imaging plates, which have largely replaced early film screens and work based on similar principles. Imaging plates are also known as flat panel X-ray detectors or flat panels.[6]
Basic Mechanisms of Imaging Plates. The main aim of the imaging plate is to take X-ray photons and convert them back into electrons, which can then be read by a digital interface. This relies on an X-ray photon-converting device and a thin-film transistor, as described below:
- X-ray Photon Conversion Device. Older electronic plates used to consist of phosphors, which are a class of compounds that light up when exposed to photon-based radiation such as X-rays.[7] Phosphors would store X-ray photons and release them as light when stimulated by an additional laser light source, which could then be converted into electrons and fed to a computer system. Since the 90s, modern imaging plates have been made that rely on modified phosphors, either scintillators or photoconductors, both of which are more efficient methods for converting X-rays into electrons. These do away with the need for an additional laser light source, do not require as much maintenance, and produce much clearer X-ray images.
- Thin-Film Transistor. A thin-film transistor (TFT) can receive the electrons from the photon conversion device and further change them into a format readable by a computer. Ions are deposited into capacitors found inside the TFT (known as detector elements), which completes parts of its internal circuitry and allows for the charge to be read out to the computer interface in a controlled manner through a TFT switch. Different types of imaging plates contain different kinds of detector elements that are used for a variety of applications.
Types of Flat Panel Detectors. There are numerous types of imaging plates available. The two main types of flat panels include direct and indirect conversion panels, which refer to the number of conversions the X-rays undergo before reaching a computer interface. These types can be further divided by the phosphors and other materials used in them, which produce unique effects and have distinct imaging applications.
- Direct Conversion Panels convert photons directly into electrons using photoconductors. Amorphous selenide is the most commonly used photoconductor, capable of converting X-rays into electrons when irradiated. This method is known to convey the highest resolution X-ray images due to its ability to separate each pixel. Despite this, direct conversion panels are not as efficient at converting high-intensity X-rays as indirect alternatives. This makes direct conversion panels better suited to low-energy applications, such as mammography, as well as for imaging superficial body tissues where resolution is important. They are typically the most expensive panel type.
- Indirect Conversion Panels convert X-ray photons to ordinary photons before converting these into electrons for the TFT to read out to the computer. Scintillators convert X-rays into photons, with the most common types being GOS (gadolinium oxysulfide; Gd2O2S) and CsI (cesium iodine). The cheaper GOS scintillators diffuse the light and often result in a more blurry image than CsI scintillators, which focus the light more and produce higher-resolution X-ray images. The light hits a photodiode comprising amorphous silicon, which then converts it into electrons before these get transferred to capacitors in the TFT. These are the most common types of panels used for general radiography purposes, and they are also the best suited for high-intensity X-ray imaging used to observe difficult-to-reach body tissues.
- CCD Indirect Conversion Panels refer to imaging plates that produce quicker, smaller X-ray images. This type makes use of CsI scintillators that feed photons into a fiber optics device. The device narrows the light as opposed to diffusing it, which is why the images generated are smaller and faster to produce. The fiber optics connect to a charge-coupled device (CCD), which converts it into electrons and feeds it to a computer interface. These are used in fluoroscopy, angiography and other applications where speed is more crucial than image size.
CT Scanning, Panel Rotation and 3D Imaging. Computerized tomography is a type of X-ray imaging technique that is capable of rendering a 3D image from X-rayed body tissue. As mentioned above, CT scanning makes use of different X-ray diffusion techniques in order to produce a flat or cone-shaped beam, which can cover a larger surface area. The imaging panel and X-ray beam emission source are positioned opposite one another on a rotating gantry, with the body tissue to be scanned resting in between them. This allows for helical scanning that produces superior, high-resolution 3D images. CT scanners have adopted indirect flat panel detectors that can handle the high-intensity X-ray radiation they produce.[8]
Fluoroscopy and Live X-Ray Imaging. Fluoroscopy is an X-ray imaging technique that makes use of multiple X-ray pulses in order to produce a video or less blurred image of moving body tissues. Aside from pulsed X-rays, its mechanism follows a similar principle as a CT scanner, making use of a C-arm with a gantry. While this may be useful, fluoroscopy is not as popular as CT scanning or MRI because it offers a lower resolution.
Spectral CT is a modified form of CT scanning. Also known as dual or multi-energy CT, it makes use of two or more X-ray beams and detectors, which collect two or more sets of imaging data. The X-rays emitted from the different sources are set at different X-ray energy intensities, allowing for precise imaging of both deeper and more superficial body compartments. This saves time and improves the resolution of both 2D and 3D images. It is currently used in angiography as a means to reduce the blurriness of cardiac imaging. It may also be employed for imaging difficult-to-reach internal body tissues (even in bariatric patients) and ailments that may not show up on conventional scans, such as brain lesions, hemorrhages, implant leaks, arthritic depositions, as well as kidney and bladder stones.[9]
Photon-Counting Detectors. Spectral CT makes use of photon-counting detectors, which can be viewed as the most advanced form of direct flat panels currently available for X-ray imaging. These detectors convert each photon into electrical signals through the use of either zinc or cadmium telluride, or silicon. Due to their specificity, they can distinguish between all radiation intensities with a very high degree of accuracy.[10]
Recent Imaging Breakthroughs and Future Directions
The below X-ray imaging developments could change the face of radiography in the next few years.
- Flexible X-ray Imaging. Up until recently, most X-ray detector panels were flat and only capable of scanning X-rays in a 2D format. A large rotating gantry has allowed for 3D imaging to take place. However, due to the expense and equipment size, inventors have been looking for 3D imaging alternatives. One such alternative would be flexible imaging panels that curve around surfaces. In the last few years, several flexible panels have been invented that improve upon all currently available flat panels (direct and indirect types) used in medical imaging devices. Some of these new inventions are able to curve around surfaces and may even be worn by the patient for comfortable scanning of selective body regions. Unfortunately, these prototypes contain heavy metals such as lead, which is likely to increase any potential radio-toxic side effects.
- Liquid Nanocrystal Imaging. In 2021, liquid nanocrystals were discovered that were capable of capturing X-rays better than currently available flat panel technology[11], allowing for optimal flexible X-ray imaging without heavy metal involvement. This technology also solves problems pertaining to irregular shapes, which often show up blurred in conventional radiographs.[12] As of yet, these liquid crystal imaging devices are not able to convert high levels of radiation into images, suggesting the course of future innovations.
- Portable X-ray Imaging Devices. With the invention of flexible panel detectors of all shapes and sizes, the possibility of portable X-ray devices is moving closer to becoming a reality. In tandem with flexibility, inventors have been working on making portable X-ray imaging devices and incorporating them into everyday gear, such as cell phones, cars, and glasses. As of 2022, an imaging plate the size of a square millimeter has been developed that can detect large quantities of photons on its minuscule surface area with a relatively high degree of accuracy.[13] With more advancements such as these, X-ray imaging devices may become as small as thermometers and integrated into the diagnostic toolkit of any general practitioner. With more time and refinement, early detection of cancer and other tissue abnormalities could be part of an everyday standard check-up.
- Tomosynthesis. An advanced type of X-ray imaging, it creates a three-dimensional image of the body. Tomosynthesis is particularly useful for detecting early signs of breast cancer as it can provide a more comprehensive view of the breast tissue, aiding in the identification of small masses or areas of concern that may be obscured in conventional mammograms. Compared to traditional 2D mammography, tomosynthesis has been shown to reduce false positives and unnecessary recalls for further testing, leading to earlier detection and potentially more successful treatment outcomes. In addition to breast cancer detection, tomosynthesis is also used in other areas of medical imaging, such as lung imaging and musculoskeletal evaluation. Its ability to offer an alternative to CT scans with lower radiation exposure in lung imaging and provide better localization of fractures and joint abnormalities in musculoskeletal evaluation makes it a useful tool in diagnosis and treatment planning.
- Improved Computation. Advances in computer software have led to better imaging as well. Algorithms have been developed that improve the accuracy of X-ray-generated images, particularly through better recovery of blurred areas and other irregularities that tend to show up on the images. However, while newer algorithms have successfully reduced artifacts (flaws in an image that are absent in the actual object captured), they remain a factor for further development since the algorithms themselves can cause artifacts. These improvements are more relevant to CT scanning. One recent development allows for highly accurate X-ray imaging of the heart by using an algorithm that incorporates the patient’s ECG results with the sensor.[14] In this way, the scanner is able to capture more accurate X-ray images of the heart from multiple angles that are matched in time to the patient’s heartbeat, thereby enhancing the resolution of the final image.
Conclusion
Through manipulating the transference of energy, X-rays can be produced and captured, allowing for precise imaging of internal body compartments. X-ray imaging has progressed tremendously since its invention in the late 1800s, shifting towards digital radiography that makes use of advanced computation to process high-resolution images. Recent developments have improved upon the selectivity and sensitivity of the equipment, enabling it to generate near-perfect imagery even involving moving body parts. Breakthroughs in imaging technology predict the future use of smaller devices with a higher degree of flexibility that allow for accurate imaging of previously undetectable microtissue problems. Future technology is expected to be less toxic as well due to lower radiation exposure and a safer material selection. In addition, advances in the use of Artificial intelligence (AI) with algorithms trained to identify specific features in X-ray images can help improve diagnostic accuracy.
To search for the best doctors and healthcare providers worldwide, please use the Mya Care search engine.
Sources:
- [1] https://www.britannica.com/science/X-ray/Fundamental-characteristics
- [2] https://chem.libretexts.org/Ancillary_Materials/Demos_Techniques_and_Experiments/Lecture_Demonstrations/Additional_Demos/Crookes'_Tube
- [3] https://www.slac.stanford.edu/pubs/beamline/25/2/25-2-assmus.pdf
- [4] https://www.ncbi.nlm.nih.gov/pmc/articles/PMC5216491/
- [5] https://howradiologyworks.com/xrayproduction/
- [6] https://howradiologyworks.com/direct-vs-indirect-digital-radiography/
- [7] https://www.britannica.com/science/phosphor
- [8] https://www.ncbi.nlm.nih.gov/pmc/articles/PMC8724686/
- [9] https://www.ncbi.nlm.nih.gov/pmc/articles/PMC4557396/
- [10] https://www.ncbi.nlm.nih.gov/pmc/articles/PMC7772378/
- [11] https://www.nature.com/articles/d41586-021-00350-2/
- [12] https://www.sciencedirect.com/science/article/abs/pii/S0022231321007055
- [13] https://www.photonics.com/Articles/Nanostructures_Advance_Machine_Vision/a68230
- [14] https://physicsworld.com/a/acrobeat-mitigates-cardiac-motion-during-imaging/