THE WORLD OF GENE EDITING AND THERAPY: NOW AND NEXT
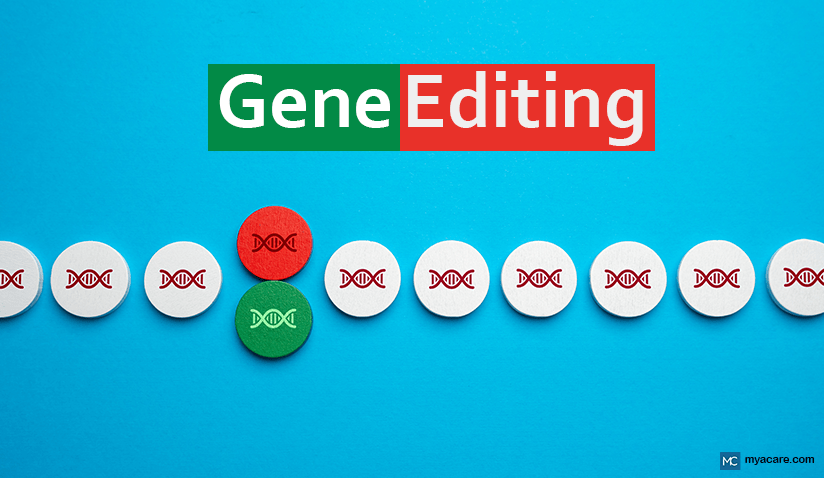
Medically Reviewed by Dr. Sony Sherpa (MBBS) - August 23, 2024
Genetics and Genetic Engineering
Purposes and Applications of Gene Editing
Gene editing is one of the most significant and controversial developments in modern science. It is the process of altering the genetic code of a living cell or organism, either by adding, removing, or modifying specific genes. Gene editing makes genetic engineering a reality, allowing for complete control over many aspects of life on the planet. From curing genetic diseases to enhancing desirable traits, the applications are endless. However, it also raises ethical and social questions about the implications of manipulating life at its most fundamental level.
In this article, we will explore what gene editing is and how genetic engineering works, its purposes and applications, as well as risks, ethical considerations, and future prospects. We will also discuss some of the recent breakthroughs and challenges in human gene editing and the impact they may have on healthcare and society.
What is Genetics? An Intro to Genetic Engineering
To understand genetic engineering and how gene editing affects our lives, it is important to have a basic understanding of genetics and how it works.
Genetics is defined as the hereditary traits of an organism or the branch of biology that deals with the study of genes and hereditary traits. Genes are segments of DNA inside every living cell that encode instructions for making proteins, which are the building blocks of life[1]. DNA (short for deoxynucleic acid) is a long molecule that consists of nucleotides. Nucleotides are molecules made of a sugar, phosphate, and a nitrogen base.
The four types of nucleotides pair up in a specific way to form DNA: adenine (A) with thymine (T) and cytosine (C) with guanine (G). This pairing gives DNA its shape as a complex double-stranded molecule (often described as a double helix) as well as the ability of the DNA to be “read” within the cell (gene expression). The sequence of nucleotides determines the order of amino acids that the cell uses as an instruction to build proteins. The complete gene sequence of an organism contains the blueprint of the organism and is called a genome.
In order to build proteins, genetic instructions are carried from genes in the nucleus to the protein-producing sites of the cell using RNA. RNA (ribonucleic acid) is similar to DNA, with the exception of being single-stranded, meaning that it comprises a single nucleotide segment and not a pair. DNA unravels and exposes one of its strands, which is copied by RNA. This is known as gene transcription.
What are Gene Mutations?
Sometimes, errors or changes occur in a DNA sequence, either due to environmental factors that lead to genetic interactions or random mutations (changes) in the original DNA sequence. These changes affect the function or expression of genes, giving rise to organismal traits that differ from the norm within a species. Many undesirable traits can cause or contribute to disease. For example, sickle cell anemia is caused by a point mutation (single nucleotide change) in the gene that codes for hemoglobin, the protein that carries oxygen in red blood cells.
What is Gene Editing?
Genetic engineering is the purposeful manipulation of genes using biotechnology. There are different techniques for genetic engineering, but two of the most common ones are:
- Gene editing involves cutting and pasting DNA at specific locations using “molecular scissors” called nucleases (enzymes that cleave nucleotides).
- Gene splicing involves joining together fragments of DNA from different sources using ligases (enzymes that create molecular bonds between nucleotides).
History of Genetic Engineering. The science of gene editing dates back to the 1950s. Since the 1980s, researchers have been trying to figure out how to correct faulty gene mutations in order to reverse chronic conditions. Early techniques were merely able to insert healthy genes into faulty genomes or leave copies of healthy genes floating around inside the cell. These strategies were only partially effective.
Scientists realized they needed to find a way to pinpoint the faulty gene in the genome (comprising of more than 3 billion nucleotide pairs), break the DNA at that point, and provide a template that allows for the cell to repair the DNA damage in a way that rewrites the DNA. It wasn’t until the invention of the CRISP-Cas9 gene editing tool that this was made wholly possible.[2]
What is CRISPR-Cas9?
CRISPR-Cas9 is currently one of the most advanced and widely used gene editing tools available. Unlike prior gene editing tools, CRISPR-Cas9 can precisely cut sections of DNA as required and allow for the introduction of new genes capable of rewriting the cell’s genetic code during the repair process. CRISPR-Cas9 is simpler, cheaper, faster and more accurate than previous technologies.[3]
How Does CRISPR-Cas9 Work?
CRISPR-Cas9 was adapted from an ancient bacterial defense mechanism against viruses. CRISPR is the abbreviation for Clustered Regularly Interspaced Short Palindromic Repeats. These are sequences of DNA that are repeated at regular intervals in some bacteria and archaea. CRISPR sequences act as a defense mechanism against viruses by storing fragments of viral DNA as spacers between the repeats. When a virus infects a bacterium or an archaeon, the CRISPR system recognizes the viral DNA and produces short RNA molecules that guide a protein called Cas9 (CRISPR-associated protein 9) to cut the viral DNA and disable it.[4]
Scientists have adapted this natural system for genome editing by engineering synthetic RNA molecules that can target any sequence of DNA in any organism. By attaching a guide RNA (gRNA) that matches the desired target sequence to Cas9 (or other variants), a CRISPR-Cas9 complex can be formed that can locate and cut the target DNA. The cut DNA can then be repaired by the cell’s own mechanisms, which can result in the deletion, insertion, or replacement of nucleotides based on the presence or absence of a template DNA.
In this way, CRISPR-Cas9 can be used to edit or splice any gene in any organism with high precision and efficiency. This can cause all cells of an organism to produce any proteins, ultimately changing its biology and the way it functions. Many iterations of CRISPR technologies have subsequently been developed since its original discovery, opening up an array of novel opportunities. Gene editing has numerous beneficial and detrimental applications depending on how it is used.
Purposes and Applications of Gene Editing
Gene editing has many potential purposes and applications in various fields of science and medicine. One of the main goals of gene editing is gene therapy, which is the use of genes to treat or prevent diseases. Other uses for gene editing extend to food production, efficient production of various biological materials, and enhanced medical research endeavors.
Gene Therapy in Medicine
Gene therapy can be somatic or germline:
- Somatic gene therapy involves modifying the genes of somatic cells, which are non-reproductive cells such as skin cells or blood cells. This is the most widely used application of gene therapy and has been used to develop treatment options for rare genetic diseases that affect specific tissues or organs.
- Germline gene therapy involves modifying the genes of germ cells (reproductive cells such as sperm or eggs) or modifying the genes of a developing embryo. Due to ethical concerns, this type of gene therapy is only used for research purposes.
Successful applications of somatic gene therapy include the invention of treatment options for cystic fibrosis, hemophilia, or muscular dystrophy. By delivering healthy copies of defective genes to the affected cells using vectors such as viruses or nanoparticles, gene therapy can restore normal function or expression of genes.
Examples of gene therapies currently approved for medical use include:
- Zynteglo for beta-thalassemia, which delivers a functional beta-globin gene into stem cells of patients with beta-thalassemia, a blood disorder that causes severe anemia.
- Zolgensma for Spinal Muscular Atrophy, which inserts a functional SMN1 gene into the motor neurons of patients with spinal muscular atrophy, a genetic disorder that causes progressive muscle weakness and paralysis.
- Luxturna for inherited retinal dystrophy, which places a healthy, corrective RPE65 gene into the retinal pigment epithelial cells of patients with inherited retinal dystrophy, a genetic condition that causes vision loss and blindness.
- Ryplazim for Congenital Plasminogen Deficiency[5], which adds the functional plasminogen gene into the liver cells of patients with congenital plasminogen deficiency, a rare genetic disorder that causes abnormal blood clotting and tissue damage.
- Yescarta, Kymriah, Tecartus, Brexucabtagene autoleucel[6] and Abecma are all examples of gene therapies that modify T cells or B cells to improve immune recognition of tumor cells. This is also known as CAR T-Cell therapy or CAR (chimeric antigen receptor) B-Cell therapy. These are being used to treat certain types of lymphoma, leukemia and multiple myeloma.
Germline gene therapy is currently being researched to prevent the genetic inheritance of diseases in future generations. By editing the genes of embryos or gametes before implantation or fertilization, germline gene therapy can eliminate harmful mutations or introduce beneficial ones. While this may seem promising, it is not yet an exact science, as revealed in early animal experiments where gene editing has led to unintentional consequences.[7]
For example, scientists attempted to use CRISPR-Cas9 to enhance muscle growth in animals by removing a gene responsible for muscle breakdown. This led to stillbirths and deformities, with unintentional and uneven muscle growth, highlighting how much has yet to be learned before this type of gene therapy can be safely implemented. [8]
Gene Editing in Food and Production Lines
Another widely used application for gene editing includes enhancing agricultural production, as well as the production of biological materials. For instance, GMO crops are already widely cultivated for their genetically enhanced resistance to drought, pests and herbicides.
For decades, gene-edited cells and bacteria have been used to create pharmaceutical drugs and therapies, including insulin derived from modified bacteria and tissue plasminogen activator derived from modified hamster ovaries. Genetically engineered bacteria have also been used successfully to make biofuels and plastics, although these are not yet being mass-produced.
In the future, animals may be genetically modified to produce more milk, meat, and wool. In America, researchers are currently looking to have engineered salmon put on the market that can double their size and halve the farming costs. Other efforts are looking at producing low-cholesterol eggs, low-fat meat and reduced lactose milk from gene-edited animals.[9]
Genetic Engineering for Improved Medical Research
Gene editing has revolutionized medical research by enabling cell and animal models of disease to be created based on genetics.
Using CRISPR technologies, genes associated with disease can now be easily inserted into embryos to produce diseased mice or in organs, tissues and cells to study them more closely.[10] Targeted therapies can then be more accurately devised to help tackle these conditions.
Modified organs from animals are also being investigated, as are growing organs from tissue cultures for organ transplantation.
Pros and Cons of Genetic Engineering
Despite its risks and ethical considerations, gene editing also has many benefits and advantages for science and society. The pros and cons of genetic engineering are discussed in brief below.
What are the Benefits of Genetic Engineering?
The benefits of gene editing include the potential to cure diseases with genetic components through gene therapies. This can also dramatically cut down treatment costs by directly enhancing the patient’s genome and dispensing with the need for future therapy. Since the majority of diseases and health conditions have a genetic component (even ordinary aging), the advantages of gene therapy extend to permanently improving healthcare, well-being, and life quality for everyone.
Other benefits of gene editing include:
- Improved agricultural practices and food security by creating crops and animals that are more productive, nutritious, or resilient.
- Rapidly advancing scientific knowledge and innovation by enabling new discoveries, applications, or products in various fields of biology, chemistry, physics, or engineering.
Risks and Ethical Considerations
Gene editing involves risks and ethical considerations. One of the principal challenges of gene editing is ensuring its safety and accuracy. Gene editing can cause unintended or off-target effects, such as damaging other genes, introducing new mutations, or activating dormant viruses. These effects can have harmful consequences for the health and well-being of the edited organisms and their offspring. For example, gene editing can cause immune reactions, infections, cancers, or genetic disorders.
Another challenge of gene editing is addressing its ethical dilemmas. Gene editing raises many moral and social questions about the implications of manipulating life at its most fundamental level. Some of these questions are:
- Who has the right to edit genes, and for what purposes?
- What are the risks and benefits of gene editing for individuals, groups, and society?
- How can we ensure that gene editing is used for good and not wrongfully?
- How can we respect the dignity and autonomy of the edited organisms and their offspring?
- How can we prevent the misuse or abuse of gene editing by individuals, corporations, or governments?
- How can we ensure that gene editing is fair and equitable for all people?
To address these questions, there are various regulatory frameworks and guidelines that govern the use of gene editing in different countries and regions.[11] These aim to balance scientific progress with ethical responsibilities and to protect the rights and interests of the stakeholders involved in gene editing. However, current legal frameworks and guidelines are not always consistent, comprehensive, or enforceable, and they often face challenges from emerging technologies, public opinions, or political agendas.
The Future of Gene Editing
As a rapidly evolving field, gene editing presents many promising opportunities and challenges for the future. With the implementation of more advanced, precise, and accessible techniques, gene editing will have more applications in various domains of science and society.
Some of the emerging fields and potential applications of gene editing are:
- Human Genome Editing: The future of gene editing is heading towards modifying human traits such as intelligence, memory, personality, or appearance. This has major implications for human enhancement, identity, and diversity.
- Synthetic Biology: Whole genomes can be fabricated from scratch, allowing for new forms of life to be created or to redesign existing organisms. An example includes incorporating spider silk genes into silkworms for the mass production of extra-strong textiles.[12] This poses serious ecological and ethical concerns that need to be carefully integrated into regulations in order to protect the environment.
- Xenotransplantation: Gene editing can be used to modify animal organs to make them compatible for human transplantation, with a lower risk of rejection or graft-versus-host disease. If ethically permissible, this will be able to cut down on waiting lists for organ donation and minimize disease transmission.
Conclusion
Gene editing is a revolutionary technology with the potential to transform our entire world. It can enable us to cure diseases, improve crops, create new products, and eventually enhance our capabilities as human organisms. However, it also poses significant challenges and risks that require careful consideration and regulation. Gene editing is not just a scientific tool but a social and ethical one as well.
As we explore the possibilities and limitations of gene editing, we need to be mindful of its impact on ourselves, our environment, and our future generations. To prevent misuse, the dignity and diversity of life in all its forms need to be respected, and the rights and interests of those who opt for gene therapy ought to be protected. With caution, appropriate regulations and testing can ensure that gene editing is used for the benefit of all people.
To search for the best Medical Genetics Doctors and Medical Genetics Healthcare Providers worldwide, please use the Mya Care search engine.
To search for the best doctors and healthcare providers worldwide, please use the Mya Care search engine.
Sources:
- [1] https://www.nigms.nih.gov/education/Documents/Studying_genes_final.pdf
- [2]https://innovativegenomics.org/education/digital-resources/what-is-crispr/
- [3] https://www.genome.gov/about-genomics/policy-issues/what-is-Genome-Editing
- [4] https://www.ncbi.nlm.nih.gov/pmc/articles/PMC8388126/
- [5] https://www.fda.gov/news-events/press-announcements/fda-approves-first-treatment-patients-plasminogen-deficiency-rare-genetic-disorder
- [6] https://www.ncbi.nlm.nih.gov/pmc/articles/PMC9526496/
- [7] https://journals.lww.com/greenjournal/fulltext/2019/07000/genetically_modified_babies_and_a_first.23.aspx
- [8] https://www.news-medical.net/news/20230626/New-study-warns-of-dangers-of-gene-editing-in-human-embryos.aspx
- [9] https://biosecurity.fas.org/education/dualuse-agriculture/2.-agricultural-biotechnology/enhanced-food-animals.html
- [10] https://mmrjournal.biomedcentral.com/articles/10.1186/s40779-023-00447-x
- [11] https://www.who.int/news/item/12-07-2021-who-issues-new-recommendations-on-human-genome-editing-for-the-advancement-of-public-health