THE EMERGING ROLES OF MAJOR NEUROTRANSMITTERS OUTSIDE OF THE NERVOUS SYSTEM: IMMUNITY, METABOLISM AND BEYOND
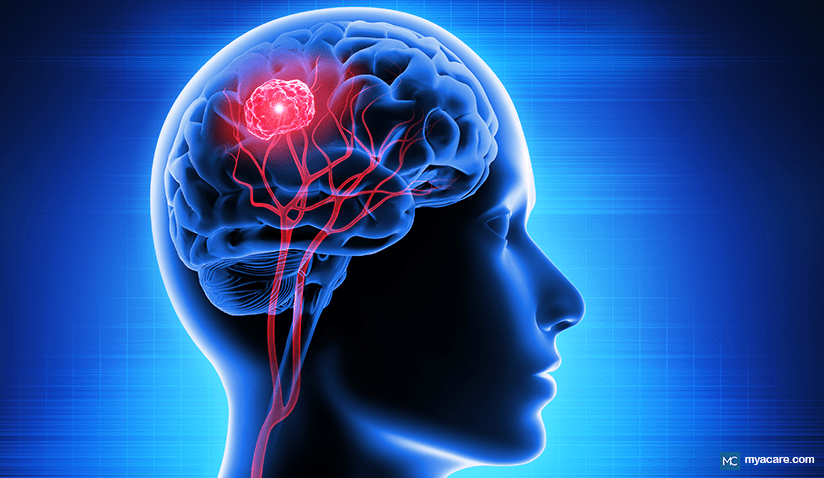
Every day, more information is published about the inner workings of our biology at the cellular level. Much of the new information that is being discovered has proven some of what we already “know” to be partially (if not fully) incorrect.
One of the areas of research coming to light in this regard would be on the functions of neurotransmitters in the body as a whole. Not all neurotransmitter functions are limited to the nervous system and secondary neuroendocrine cells as previously thought. In fact, many ordinary cells appear to possess the ability to manufacture neurotransmitters, as well as having receptors that are able to receive them in way different to neurons and neuro-endocrine cells. Ordinary cells have been shown to respond directly to neurotransmitters, using them in many vital cellular processes which are only now beginning to be highlighted through research.
These discoveries are profound in the sense that they have the potential to change the way treatments are designed for patients with both neurological and non-neurological diseases. Many pharmaceutical drugs previously thought to interact purely within the nervous system will now have to be reconsidered for the way they may interfere with neurotransmitter signaling outside of the nervous system.
The following article attempts to briefly review what is known about non-neuronal neurotransmitter signaling and shed some light on how that may affect the future of medication moving forward. Five major neurotransmitters are discussed in this regard, revealing other body sites in which they are active and why.
Neurotransmitters Are Active Agents Before the Arrival of a Nervous System in Utero
Perhaps one of the most literal ‘mind-boggling’ pieces of research to surface in this field of biology would be the observation of neurotransmitter activity in the developing embryo.
After fertilization has occurred, the embryo is hard at work dividing and growing in order to become a fully-fledged fetus. On day 8 of this process, neurotransmitters can be seen functioning in the absence of a nervous system alongside neurotropic factors. These substances are released from the amniotic membrane surrounding the embryo and appear to be involved in promoting the development of the nervous system. This was observed to be the case for both human and monkey embryos[1].
This casts a shadow of doubt on the true purpose of neurotransmitters and whether their primary functions are purely neurological or if they are more like ‘cellular-transmitters.’
Catecholamines Are Adrenal Neurotransmitters with Hormonal Actions
Catecholamines are perhaps the most famous neurotransmitters to act as hormones in non-neuronal tissues. They are produced in the adrenal glands and work along the hypothalamic-pituitary-adrenal axis to reach the adrenergic centers of the brain in the nervous system, constituting the majority of autonomic nervous activity. Main catecholamines include epinephrine, norepinephrine, acetylcholine and dopamine.[2]
Outside of the nervous system, catecholamines are able to activate receptors in smooth muscle cells which then cause the muscles to contract[3]. This is still within the realm of the nervous system however as smooth muscle cells are not typically known to produce neurotransmitters. A growing body of evidence reveals that they may be capable in some instances, as discussed below.
Novel Non-Neuronal Functions of 5 Major Neurotransmitters
Many non-neuronal cells also appear to harbor the ability to produce, store, release, and receive neurotransmitters. Some of the cells even possess similar neurotransmitter signaling mechanisms to that of neurons. Examples of such neurotransmitters include glutamate, GABA, dopamine, serotonin and acetylcholine.
(1) Glutamate
Glutamate is one of the most well-known of the excitatory neurotransmitters and it tends to be the main one involved in the vast majority of nerve transmission.
Multiple bodily sites outside of the nervous system appear to harbor the ability to store, transmit and receive glutamate in similar ways to that of neurons[4]. Aside from the many areas listed below, glutamate receptors have been noted in the spleen, stomach, adrenal glands, pituitary gland, pineal gland, ovaries, and lymph glands, yet their functions in these areas are still largely unexplored.
The following sites have been shown to use glutamate independently of the nervous system for a variety of important cellular functions:
- Bone
Glutamate expressed in bone tissue seems to promote the growth of bone cells as well as their differentiation, depending on which receptors are activated. Different types of bone cells convey different types of glutamate receptors with unique functions. If glutamate accumulates in and overloads bone cells, it inhibits their growth and differentiation.
While glutamate appears to play a large role in regulating bone cell turnover, little more is known about the process. Bone cells and their membranes are able to transport glutamate in a similar fashion to neurons and release this neurotransmitter into the extracellular space so that other cells can receive it as a signal.
They also appear to possess all the right components in order to produce their own glutamate, yet whether they are capable of doing so is a mystery[5]. Nerve cells tend to proliferate near bone cells that are capable of releasing glutamate and are affected by bone cell signals in this manner. In this sense, it is unclear if the source of glutamate is the nervous system or the bone cells themselves and where this chemical cross-talk began.
- Testis
Rat experiments reveal that the testis have several glutamate receptors and are able to respond to this neurotransmitter and its analogues. The way the testis respond to glutamate directly, however, is unclear. Using an analogue (D-aspartate) to block glutamate receptors in Leydig cells caused them to generate larger amounts of testosterone. This may prove to be useful information in treating certain reproductive disorders where too much testosterone causes the prostate to become enlarged.
- Pancreas
In test-tube studies, it has been demonstrated that the beta cells, comprising the islets of Langerhans in the pancreas, all have several receptors for receiving glutamate. When glutamate binds to the receptors of these cells, they secrete more insulin and glucagon. Furthermore, a specific glutamate receptor becomes active when high levels of glucose are present and this results in a regulatory inhibition of both insulin and glucagon release. In this way, glutamate is involved in the regulation of glucose metabolism.
As beta cells have the ability to store and release glutamate, it is likely that they are capable of producing their own supply of the neurotransmitter. However, it remains to be seen whether this is the case. A few neuroendocrine cells in the pancreas have been proven to produce and release glutamate. In low glucose conditions, these cells release glutamate which becomes converted into GABA (an inhibitory neurotransmitter) in beta cells, therefore inhibiting glutamate signaling.
Various types of white blood cells have the ability to respond to glutamate signaling. Mast cells, antigen presenting cells (especially dendritic cells) and T cells are the most extensively studied immune cells with regard to glutamate signaling.
It is difficult to generalize as there are many types of glutamate receptors and the response of immune cells to this neurotransmitter vary depending on what receptors they possess, the concentration, and what other signaling molecules present alongside it. In general, low to normal concentrations of glutamate appears to promote the expansion of white blood cells, particularly T cells. In very high concentrations, it seems to inhibit the proliferation and cytokine expression of these cells.[6]
The above findings may prove beneficial for treating various immune-related diseases as inhibiting or upregulating glutamine may be able to boost or tone down the immune response as required.[7]
- Heart
Excessive glutamate signaling in the muscle tissue of the heart has been shown to contribute towards disease-related changes seen in pulmonary arterial hypertension.[8] These changes include stiffening of the blood vessels as well as vascular cells that continue to grow and are resistant to dying off. This was observed to arise with altered energy metabolism in these cells that favors oxidative stress.
- Blood Platelets
Also referred to as thrombocytes, blood platelets are the smallest cells present in the bloodstream. They are involved in blood clotting or thickening of the blood in the event of bleeding[9]. Interestingly, they are also cells that appear to have many receptors for major neurotransmitters, including all the ones discussed in this section and below. It is not fully understood why platelets are endowed with the ability to store, release and respond to neurotransmitters such as glutamate.
In the case of glutamate, the neurotransmitter appears to activate blood platelets and promotes their blood-clotting activities[10]. This may be either beneficial or detrimental depending on the circumstance.
For example, glutamate excesses can cause neurons to die off and as described in this section, other cells to change metabolism in a way that is detrimental. However, a study recording the effects of glutamate on platelets in animal models of ischemic stroke showed that this glutamate-releasing ability of platelets enhanced their protective actions. The neurons experiencing the ischemic damage expressed increased glutamate levels and receptors which ultimately is a recipe for neuronal death. Platelets helped to lower the expression of a specific glutamate receptor on the membrane of the neurons, which promoted their survival and prevented cell death as a result of glutamate-induced excitotoxicity.[11]
Other studies reveal that glutamate-induced platelet activation actually contributes towards the onset and progression of migraines in some cases.[12]
- Lungs
Multiple types of glutamate receptors have been found in the lungs, however it is still not entirely clear why. Studies reveal that the activation of specific glutamate receptors (NMDA receptors) in lung cells can induce lung injury, swelling and pulmonary edema[13].
These receptors also become active when the lung is inflicted with an acute injury. In animal studies, blocking these receptors completely prevented symptoms of sepsis-related lung damage in response to an injury[14].
- Kidneys
An interesting series of observations regarding glutamate signaling in the kidneys may change the way the kidneys are known to function as a whole. Glutamate appears to be an integral part of kidney filtration. The kidney cells that make up the glomerulus (podocytes) have been shown to have glutamate receptors, the ability to produce and release glutamate as well as respond to glutaminergic signals in an identical way to neurons.[15]
Glutamate signaling helps to control and regulate protein filtration. If the signaling is unbalanced, it tends to create increased stiffness of the delicate arteries in the kidneys and can increase the risk of kidney disease. Too much activation of glutamate receptors tends to cause a buildup of proteins in the blood (specifically homocysteine), while too little activation can cause too many proteins to leave the body (specifically albumin)[16].
- Skin
While not a lot is known about glutamate signaling in skin, multiple receptors have been found in skin cells (keratinocytes) as well as the ability for glutamate transport.[17]
Evidence supports the notion that glutamate enhances wound healing and repair in damaged skin. When coupled with glutamate signaling in blood platelets and the increase in neurologic activity in response to the skin wounds, it would make sense that the skin and other sites might respond to glutamate in this fashion.
In embryonic development, glutamate also appears involved in the formation and growth of fetal skin in the absence of a wound.
- Tumor Formation
Certain types of tumors, such as lung tumors, have been shown to develop a fully functioning glutamate signaling system of their own in order to function.[18] Tumor glutamate appears to promote the growth and expansion of the tumor, and tends to increase levels of oxidative stress and inflammation.
Lung tumors have been studied with specific regard to glutamate signaling, with research revealing glutamate and glutamine as being highly implicated as an alternative energy source to glucose for the tumor. They are also involved in the production of proteins that the tumor then uses to grow.[19]
Further research is required in order to assess the potential implications of this evidence moving forward.
- Antioxidant Defense
Perhaps one clue alluding to the diverse applications of glutamate throughout the body would be its propensity to be transformed into a major cellular antioxidant. Glutathione is an antioxidant that is produced by the mitochondria in every cell of the body. This antioxidant is composed of cysteine, glutamate and glycine (a glutamate precursor). Further research reveals that glutamate can be produced by the mitochondria as well.[20]
Studies on neuronal glutathione have shown that it is in fact a valuable source of glutamate storage in the nervous system[21]. However it’s use in the formation of glutathione suggest a far greater role for glutamate in the body as a whole. Glutathione is used to combat nearly all forms of cellular stress and helps to ameliorate oxidative stress. Oxidative stress is detrimental in high levels as well as being a natural part of mitochondrial function, serving as a different type of cell signal depending on the intensity or volume of the oxidation.
When the enzyme used for converting glutamate into glutathione is inhibited or when there is not enough available cysteine, glutamate begins to build up in the nervous system. This consequently induces high levels of oxidative stress and neuronal cell death. If the same can be said of other cells in the body in relation to glutathione production, then it suggests that over-excitability of the nervous system and the resultant cross-talk between non-neuronal cells may cause glutathione to decrease and oxidation to increase in other areas of the body due to an increase in glutamate release.
Glutathione deficiency is a very common component of inflammatory diseases and this discovery adds to the far-reaching implications of glutamatergic signaling in states of disease.
(2) GABA
GABA is glutamate’s equal opposite neurotransmitter, serving an inhibitory function over the actions of glutamate[22]. Since GABA is synthesized from glutamate when in the presence of Vitamin B6 and a few enzymes, it logically follows that wherever glutamate is operating, GABA is not far behind and serving to regulate glutamate.
Unfortunately, while GABA and its receptors have been found in many peripheral bodily tissues outside the nervous system[23], these sites of GABA transmission have not been studied as well as other neurotransmitters. Aside from the sites listed below, GABA is also found in the lungs[24], heart, blood vessels and stomach, however their presence in these areas is a mystery.
- Immune System
GABA signaling is fully functional in many immune cells outside of the nervous system[25], including macrophages, T lymphocytes (T cells) and antigen presenting cells.
In most cases, GABA has an inflammation-lowering effect on the immune system. It has been shown to lower the severity of allergic immune responses (delayed-type hypersensitivity reactions) as well as decrease the intensity of autoimmune responses in reactive T cells[26].
Recently it was shown in experimental models of multiple sclerosis, an autoimmune disease, that GABA signaling may prevent immune cells from creating auto-antibodies against the myelin sheath.[27]
- Blood Platelets
GABA appears to play a similar role in blood platelets to that of glutamate, promoting their aggregating abilities in a calcium-dependent manner.[28]
A very bizarre finding highlights the roles of both GABA and glutamate in the blood platelets of those with bipolar disorder. It seems that during the manic (euphoric) phase of bipolar disorder, the blood platelets of the patient take up excessive amounts of glutamate. During the depressive phase, the platelets appear to take up significantly increased levels of GABA.[29]
It is unknown whether this is enough to alter brain chemistry or GABA signaling in other areas of the body, however it does seem to parallel the dual nature of the condition. Scientists speculate that blood platelets will be used in future to accurately diagnose the active phase of disease in bipolar patients.
- Pancreas
The pancreas was one of the first sites known to have a GABA signaling system that is independent of the nervous system[30]. For many decades it was a mystery as to why beta cells in the pancreas store and utilize GABA in a similar fashion to that of neurons.
After many studies, it was revealed that GABA is released when glucose and insulin levels are low. When glucose and subsequent insulin release increases, the levels of GABA drop. This makes sense in the context of glutamate, which tends to increase insulin release and is inhibited by the actions of GABA. It also suggests that GABA helps to regulate insulin release by inhibiting it when levels become too high.
GABA may also be able to regulate glucagon by lowering its expression when docking in at certain GABA receptors in the pancreas. However, more evidence is required still to prove this observation as fact.
This inhibitory neurotransmitter may play a central role in regulating growth of the cells in the pancreas.[31] Low GABA concentrations were shown to induce pancreatic hyperplasia (overgrowth) in the early stages of diabetes and it is observed that GABA is downregulate in diabetic subjects[32]. An enzyme that converts glutamate into GABA (GAD 65) is also a main target for the autoimmune attack seen in those with diabetes that ultimately leads to the complete destruction of the pancreas, further adding to this hypothesis.
Interestingly, administration of GABA or GABA receptor agonists were shown to improve parameters of diabetes in experimental models by completely regulating pancreatic hormone secretion[33].
- Kidney
It has been shown that the kidneys express many kinds of GABA receptors and that GABA may be involved in regulating bodily water and sodium balance. Increased GABA signaling in the kidneys has been shown to promote sodium excretion and lower blood pressure as a result[34]. This may also be suggestive of a potassium sparing effect, but more research would be required to confirm if that is the case.
Interestingly, certain types of GABA receptors are only temporarily expressed in the kidneys of rats. This suggests that GABA receptors may have been missed when looking for their appearance in non-neuronal tissues[35]. Furthermore, the GABA receptors that express in kidney cells appear to counteract the negative effects of homocysteine when present in very high concentrations (hyperhomocysteinemia). As this is a feature of several pathologies, including kidney diseases, there may be some benefit in designing medications that promote and target GABA receptors.
- Gums and Teeth
A surprising study revealed that GABA receptors and signaling exist in the gums, particularly in the layer of the gums that holds the teeth in place[36]. It is not known what GABA is doing here, however it is known that inflammation and the state of the immune system changes the expression of specific GABA receptors. This suggests that GABA is involved in either maintaining or regulating the immune response in the gums.
(3) Dopamine
Dopamine is the major neurotransmitter associated with reward, motivation and pre-meditated movements[37]. As a result, it is also released within the context of learning, memory and positive emotions.
While dopamine is known to be made in the brain and adrenal glands, it is synthesized and utilized as a hormone in a few non-neural cells.
- Immune System
Dopamine can be released by immune cells and moderates the immune response. In T lymphocytes, dopamine promotes an inflammatory response, shaped by a Th1 or Th17 response. Moreover, dopamine inhibits the expansion of regulatory T cells, which further promotes an inflammatory response[38]. In this context, dopamine excess may play a role in exacerbating symptoms in the context of autoimmune conditions.[39] [40]
- Heart and Blood Vessels
At low levels, dopamine has been shown to induce vasodilation in all blood vessels and to increase the heart rate. At higher levels, it can cause the opposite effect, constricting blood vessels.[41]
Animal studies reveal that dopamine stores are greater in the blood vessels of the kidney and the mesenteric artery[42]. The mesenteric artery supplies a large portion of the gut with blood flow and tends to pose a large degree of vascular resistance to blood flow. Extra dopamine stores in this part of the vascular system suggest that dopamine may be used in order to modulate the contractility of the blood vessels in this region in order to overcome this resistance as required.
- Blood Platelets
Blood platelet aggregation may be either inhibited or enhanced by the effects of dopamine. In low concentrations, dopamine has minimal effects on platelet aggregation, however due to its serotonin-lowering effects, it tends to decrease aggregation. When concentrations become high enough, dopamine stimulate aggregation and can even promote over-aggregation.[43]
In Parkinson’s disease[44] and certain conditions such as paraganglioma[45], blood platelets have shown to carry much larger amounts of dopamine than seen in healthy individuals. This suggests a role for dopamine transmission from blood platelets in these pathologies.
- Kidneys
The kidneys are one of the best studied bodily regions of non-neural dopamine expression[46]. Dopamine is produced, stored and released locally in the kidneys, alongside the expression of a few of its receptors. Like GABA, Dopamine enhances sodium excretion in the urine and thus helps to lower blood pressure. The level of dopamine release and receptor expression increases when dietary sodium intake increases or when intracellular sodium concentrations rise.[47]
In spite of this, more research is still required before it can be confirmed that dopamine helps with hypertension.[48]
- Pancreas
Like other neurotransmitters, beta cells in the islets of the pancreas are capable of synthesizing and using dopamine. Dopamine appears to play an important role in regulating insulin secretion, able to lower its expression in pancreatic cells. However, when dopamine reaches very high levels, it appears to promote insulin secretion.[49]
(4) Serotonin
Serotonin is a major neurotransmitter that also serves as a major cellular signaling molecule across many cell types. While much research has focused on its role in the nervous system, the rest of the entire body uses serotonin as a hormone. Primitive organisms (dating back over 500 000 years ago) and embryonic cells that do not possess a nervous system also make use of serotonin as a hormone.
Functions for serotonin have been established in the following tissues, however these findings suggest that it is likely that most, if not all, cells use serotonin.
- Digestive Tract
Approximately 90-95% of all the serotonin in the body is produced and secreted by enterochromaffin cells in the gastrointestinal tract.[50] This local serotonin helps to regulate digestive functions including peristalsis, digestive secretions, physical sensations and inflammation. It also counts as a separate type of serotonin than the one produced by the nervous system and is received differently by cells in the body as a whole.
Blood platelets take up serotonin from the gut and transport it to other areas of the body, including the bone and liver.[51] If serotonin synthesis is low in the gut, it results in reduced motility and cellular turnover[52], as well as a lowered capacity for bodily regeneration.
- Bone
Bone cells have the ability to synthesize, store and release serotonin in a similar way to neurons[53]. Interestingly, not much is known about local serotonin synthesis in bone, however serotonin from other sites of the body have opposing effects on bone cells. If serotonin is expressed from the brain via peripheral nerves into bone tissue, it promotes bone growth and cellular turnover. If serotonin made in the gut finds its way to the bone, it inhibits bone growth. [54]
- Pancreatic Function
It was found that serotonin also plays a major role regarding insulin secretion in the pancreas. Mice that genetically lacked the cellular ability to produce serotonin were shown to have the defining features of diabetes. These mice were also non-responsive to an anti-diabetic drug that would usually promote insulin release. When treated with serotonin, the pancreatic beta cells in the mice began to secrete insulin and normalize their glucose levels.[55]
- Immune System
Serotonin has been shown to regulate immune function and can be produced by mast cells, macrophages and T lymphocytes.[56]
In pro-inflammatory conditions, it serves to maintain the balance between inflammatory and anti-inflammatory immune cells. In other studies, it helped to increase inflammatory immune signaling and enhance the immune response in specific white blood cells (natural killer cells). Under inflammatory conditions, serotonin helps to prevent immune cells from dying off in the cross-fire.
The effects of serotonin in the context of immunity are highlighted in diseases of the gut such as irritable bowel syndrome, crohn’s disease and celiac disease. In many of these diseases, serotonin levels in the gut are greatly elevated due to the over-activity of enterochromaffin cells. It has also been shown that this elevation correlates with digestive issues and increased inflammation in the gut. This implies that while serotonin may regulate immune processes in other areas of the body, it is not helpful in the gut where it is already produced in mass.
- Blood Platelets
Unlike the other neurotransmitters that platelets absorb, store and release, serotonin is the number one marker to show that platelets are active when testing blood samples. At the site of an injury, blood platelets release serotonin which quickly causes them to aggregate and exudes a vasoconstrictive effect, which helps to minimize bleeding.[57] Adding to this regenerative effect, serotonin appears to enhance the growth of smooth muscle tissue as well as blood vessels.
Over-expression of serotonin from blood platelets is known to exacerbate asthma, sepsis and thrombosis; the latter of which can promote the onset of a heart attack or stroke.[58]
(5) Acetylcholine
While Acetylcholine plays a fundamental role in nerve conductance, it is far more than just a major neurotransmitter. Plenty of research exists to show that acetylcholine is produced by nearly all living cells in nearly all living organisms on the planet, including humans, animals, plants, microbes and other simplistic organisms. Nearly all types of cell in the human body possess the ability to produce and release acetylcholine, as well as the receptors to receive it.[59]
There are two main types of acetylcholine receptors: muscarinic and nicotinic. These two types are divided into multiple subtypes, adding up to more than 20 receptor types in total. Each specific receptor can be modulated by the presence of acetylcholine in conjunction with another major signaling chemical, such as a hormone, cytokine, growth factor, peptides, etc. In this way, the chemistry governing the functions of non-neural acetylcholine is very complex and gives rise to a large number of configurations that in differentially regulate the cell it acts upon.
The most consistent function of acetylcholine across all cell types would be regulating the growth, adhesion and turnover of cells. As acetylcholine performs many important functions throughout the body, only a few are discussed below to highlight its importance.
- Skin, Muscle and Bone Cells
The functions of acetylcholine are similar in all types of skin cells, from the cells that house the body to the internal skin cells that house organs and other tissues. Depending on the receptor and level of other signaling molecules present, acetylcholine promotes skin growth, cell adhesion, cell turnover, and regeneration.
In muscle tissue, it has been shown that acetylcholine performs similar functions to that of skin. It promotes the growth and development of blood vessels, muscle tissue and the release of nitric oxide, which is a vasodilator and cellular signal in its own right.
Acetylcholine also appears to regulate bone growth and adhesion in a similar fashion, promoting the formation of strong, healthy bone cells.[60]
- Immune System
In immune cell lines, acetylcholine serves a regulatory role. It lowers inflammation, promotes immune cell growth, and increases both inflammatory and anti-inflammatory cytokine production. In some models of allergy, acetylcholine proved to reduce the severity of the immune reaction by promoting the expansion of regulatory T lymphocytes.
It also helps to ensure that antibody production does not get out of hand in B lymphocytes, which suggests it may be beneficial for those with autoimmune conditions.
- Pancreas
Like many of the neurotransmitters discussed above, acetylcholine regulates insulin secretion in the beta cells of the pancreas. Further research demonstrates that it also maintains somatostatin release in the pancreas, which further regulates insulin secretions.[61]
- Blood Platelets
Unlike all other neurotransmitters discussed, acetylcholine is the only one that is able to inhibit the aggregation of blood platelets[62]. This translates to thinning the blood and increasing the risk of bleeding. On the positive side, it can help protect against thrombosis or excessive blood clotting, which increases the risk of stroke.
Conclusion
As evidenced by the above discussion, neurotransmitters appear to have many diverse functions outside of the nervous system and may need to be renamed to ‘cellular transmitters.’
Well-studied non-neuronal cells that make use of neurotransmitters include blood platelets, pancreatic beta cells, bone cells, and white blood cells. Kidney cells, vascular cells, heart cells, skin cells and muscle cells are also known to use neurotransmitters to lesser degrees for a variety of functions.
Every cell in the body makes use of acetylcholine. This particular neurotransmitter appears to be involved in regulating the growth of many organisms at the cellular level.
The above findings suggest that neurotransmitters may be used in the treatment of various diseases, especially autoimmune, neurodegenerative, cardiovascular, and osteoporotic conditions. Further research is required to outline the interactions of currently existing treatments with neurotransmitter systems in the body as a whole in order to improve health outcomes.
To search for the best Neurology Healthcare Providers in Croatia, Germany, India, Malaysia, Spain, Thailand, Turkey, Ukraine, the UAE, UK and the USA, please use the Mya Care search engine.
Sources:
- [1] https://pubmed.ncbi.nlm.nih.gov/11243569/
- [2] https://www.ncbi.nlm.nih.gov/books/NBK507716/
- [3] https://www.ncbi.nlm.nih.gov/pmc/articles/PMC3139802/
- [4] https://febs.onlinelibrary.wiley.com/doi/full/10.1046/j.1432-1033.2003.03907.x
- [5] https://www.ncbi.nlm.nih.gov/pmc/articles/PMC3412269/
- [6] https://pubmed.ncbi.nlm.nih.gov/24584970/
- [7] https://www.ncbi.nlm.nih.gov/pmc/articles/PMC2997749/
- [8] https://www.ncbi.nlm.nih.gov/pmc/articles/PMC8113389/
- [9] https://www.britannica.com/science/blood-biochemistry/Platelets-thrombocytes
- [10] https://www.ncbi.nlm.nih.gov/pmc/articles/PMC2275381/
- [11] https://pubmed.ncbi.nlm.nih.gov/24745710/
- [12] https://pubmed.ncbi.nlm.nih.gov/17212681/
- [13] https://pubmed.ncbi.nlm.nih.gov/17344592/
- [14] https://www.ncbi.nlm.nih.gov/pmc/articles/PMC5938426/
- [15] https://pubmed.ncbi.nlm.nih.gov/16585060/
- [16] https://pubmed.ncbi.nlm.nih.gov/25829324/
- [17] https://pubmed.ncbi.nlm.nih.gov/10084312/
- [18] https://pubmed.ncbi.nlm.nih.gov/16307489/
- [19] https://pubmed.ncbi.nlm.nih.gov/30634602/
- [20] https://www.ncbi.nlm.nih.gov/pmc/articles/PMC2217445/
- [21] https://pubmed.ncbi.nlm.nih.gov/21539809/
- [22] https://www.ncbi.nlm.nih.gov/books/NBK526124/
- [23] https://pubmed.ncbi.nlm.nih.gov/2999544/
- [24] https://pubmed.ncbi.nlm.nih.gov/23568330/
- [25] https://pubmed.ncbi.nlm.nih.gov/25677654/
- [26] https://pubmed.ncbi.nlm.nih.gov/29627388/
- [27] https://www.pnas.org/content/107/6/2580
- [28] https://www.researchgate.net/publication/26700091_Investigating_GABA_and_its_function_in_platelets_as_compared_to_neurons
- [29] https://pubmed.ncbi.nlm.nih.gov/22548903/
- [30] https://www.ncbi.nlm.nih.gov/pmc/articles/PMC2217445/
- [31] https://pubmed.ncbi.nlm.nih.gov/28280900/
- [32] https://pubmed.ncbi.nlm.nih.gov/22538358/
- [33] https://pubmed.ncbi.nlm.nih.gov/34084316/
- [34] https://www.ncbi.nlm.nih.gov/pmc/articles/PMC4154856/
- [35] https://www.ncbi.nlm.nih.gov/pmc/articles/PMC3182488/
- [36] https://pubmed.ncbi.nlm.nih.gov/26865191/
- [37] https://www.ncbi.nlm.nih.gov/pmc/articles/PMC4684895/
- [38] https://www.ncbi.nlm.nih.gov/pmc/articles/PMC5863511/
- [39] https://www.frontiersin.org/articles/10.3389/fimmu.2014.00117/full
- [40] https://link.springer.com/article/10.1007/s11481-019-09851-4
- [41] https://www.ncbi.nlm.nih.gov/books/NBK535451/
- [42] https://pubmed.ncbi.nlm.nih.gov/3931730/
- [43] https://pubmed.ncbi.nlm.nih.gov/1382907/
- [44] https://www.ncbi.nlm.nih.gov/pmc/articles/PMC1702717/
- [45] https://pubmed.ncbi.nlm.nih.gov/26677887/
- [46] https://pubmed.ncbi.nlm.nih.gov/16106242/
- [47] https://www.ncbi.nlm.nih.gov/pmc/articles/PMC4154856/
- [48] https://pubmed.ncbi.nlm.nih.gov/7642168/
- [49] https://pubmed.ncbi.nlm.nih.gov/22918877/
- [50] https://www.ncbi.nlm.nih.gov/pmc/articles/PMC5346549/
- [51] https://www.ncbi.nlm.nih.gov/pmc/articles/PMC5526216/
- [52] https://pubmed.ncbi.nlm.nih.gov/31071306/
- [53] https://pubmed.ncbi.nlm.nih.gov/29101650/
- [54] https://pubmed.ncbi.nlm.nih.gov/20921133/
- [55] https://journals.plos.org/plosbiology/article?id=10.1371%2Fjournal.pbio.1000227
- [56] https://www.ncbi.nlm.nih.gov/pmc/articles/PMC5526216/
- [57] https://www.ncbi.nlm.nih.gov/pmc/articles/PMC5346549/
- [58] https://www.intechopen.com/books/serotonin-a-chemical-messenger-between-all-types-of-living-cells/immuno-thrombotic-effects-of-platelet-serotonin
- [59] https://www.ncbi.nlm.nih.gov/pmc/articles/PMC2518461/
- [60] https://pubmed.ncbi.nlm.nih.gov/32208165/
- [61] https://www.ncbi.nlm.nih.gov/pmc/articles/PMC4113066/
- [62] https://pubmed.ncbi.nlm.nih.gov/30765424/
Disclaimer: Please note that Mya Care does not provide medical advice, diagnosis, or treatment. The information provided is not intended to replace the care or advice of a qualified healthcare professional. Always consult your doctor for all diagnoses, treatments, and cures for any diseases or conditions, as well as before changing your healthcare regimen. Do not reproduce, copy, reformat, publish, distribute, upload, post, transmit, transfer in any manner or sell any of the materials in this blog without prior written permission from myacare.com.